[Originally published as the second part of Quantum Particles: An Introduction]
Quantum physics deals with how the universe behaves at very small scales — on the level of atoms and smaller. And it’s weird: very weird. Particles that are smaller than atoms do not behave in exactly the same way as the much larger objects to which we are accustomed. This makes quantum physics one of the most intriguing areas of research and one which declares the glory and majesty of the Lord.
The Wave Nature of Matter
But why are electron levels quantized? The answer concerns the wave nature of matter. Early in the history of physics, there was considerable debate about whether light was a wave or comprised of individual particles. We now know that light is (in a sense) both. It sometimes behaves like a wave, and other times it behaves as if it were made of particles.
At first glance, this might seem contradictory because a wave is extended in space, whereas a particle has only a single location in space and is not extended.
Consider dropping a rock into a lake. The splash will form a ripple pattern of concentric circles that expands with time. If the splash is large enough, the ripples might eventually fill the entire lake. So if we ask, “Where is the ripple?” The answer is, “Everywhere — it fills the surface of the lake.” But a single particle of infinitesimal size cannot do that. It exists at only one specific location in space at any given time.
Through a variety of experiments, physicists have discovered that light, electrons, and in fact, all particles have a wave nature. Under certain circumstances, they act as if they are a wave that fills a certain volume of space. At other times, they behave as if they are particles, existing at only one specific location in space. However, quantum particles never behave as both a wave and a particle at the same time. Thus, there is no contradiction.¹
So, when do quantum objects act like particles, and when do they act like waves? The answer is apparently that they act like particles when they are observed and like waves when they are not observed. This seems very peculiar for multiple reasons.
- How does the particle know it is being observed so as to change its behavior?
- How do we know it’s a wave when we are not observing it?
As to the first question, keep in mind that we cannot observe a quantum particle without affecting it. We tend to think of observation as passive. If we want to observe something large, we just shine a light on it, and the reflected light enters our eyes and informs us what the object looks like. Shining light on a large object doesn’t affect it very much because the object is so much more massive than the particles of light that impact it. But in the quantum world, particles are so small that anything we use to observe them will have a significant effect on the particle.
The Two-Slit Experiment
To answer the second question, we know that quantum objects behave like waves when we are not observing them because of where the particles end up. This was demonstrated in the famous two-slit experiment. In this experiment, there is a light source some distance from a wall. The wall has two narrow, vertical slits in it. Light can pass through the slits. These slits are microscopic — separated by a distance comparable to the wavelength of light. Another wall exists behind the first and records where the light strikes.
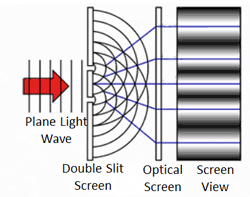
We know how waves behave in this situation from our observations of large-scale waves (such as waves in water). A wave emitted from a source will impact the wall, but sections of the wave will pass through both narrow slits — forming two new waves that move away from the slits. The two waves begin to overlap as they move toward the second wall.
In some places, the peak of one wave overlaps with the trough of the other, and the two opposites cancel out. In other places the peak of one wave adds to the peak of another, forming a really tall peak. Thus, there are certain places on the second wall where the waves are very strong (because the two sources reinforce each other) and other places where the waves are non-existent (because the two sources have canceled out). This is called an interference pattern.
We can compute the interference pattern based on the wavelength of light.
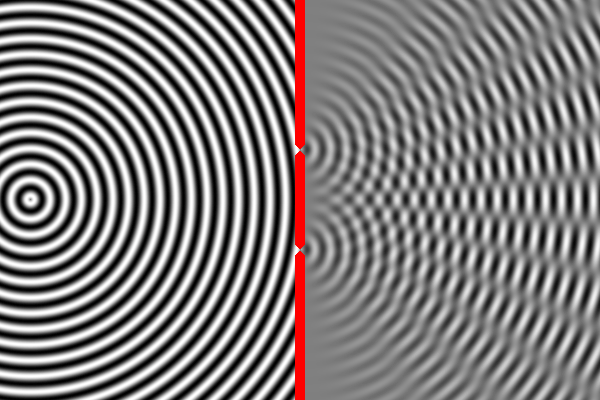
Sure enough, the two-slit experiment confirms that light is a wave. Send light of a given wavelength toward the slits, and the wave will go through both, forming two new waves which create an interference pattern. Yet, we also know that there is a minimum energy level for light of a given wavelength. You can have one unit of energy, or two, or three, but not 1.5 or one-half, or one-quarter. This suggests that light is made of indivisible particles — each unit of energy corresponding to one particle. These particles are called photons.
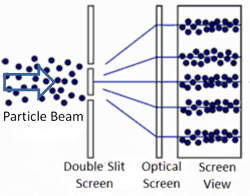
So what happens when we do the two-slit experiment, sending the light in one photon at a time? The answer is that the photon will always land on one of the places where the two waves combine constructively and never on the places where the two waves cancel out. In other words, the photon has apparently traveled as a wave (extended in space) and has gone through both slits, forming two waves that interfere with each other.
Only when these waves strike the wall does the photon again act like a single particle at one location in space. And the location it picks is always where the waves are strongest. It’s amazing and counterintuitive. But this experiment has been repeated many times, and this is always the result.
The equation describing the peaks and troughs of the wave is called the wave function. Scientists have discovered that the square of the wave function is exactly equal to the probability of detecting the particle at that location. So that is why the photon always ends up where the waves are strongest and never where they cancel out.
Apparently, one photon — a single particle of light — can go through both slits because it behaves like a wave. It is extended in space and can therefore pass through both slits, forming two new waves that interfere with each other. Yet, our intuition suggests that the particle can only pass through one slit. So it is tempting to put a detector on the slits to observe which slit the photon passed through. We actually only need a detector on one of the two slits because if it does not detect the photon, then we know it must have gone through the other slit. So, what happens when we place a detector on one of the two slits?
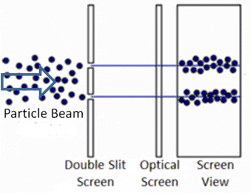
The two-slit experiment has also been performed with electrons. They also form an interference pattern, even when the electrons are sent through the slits one at a time. All particles have a wave function. The wavelength decreases with increasing energy, which is why we cannot detect the wave function of macroscopic objects.²
Footnotes
- A contradiction is “A and not-A at the same time and in the same sense.” An electron sometimes behaves as a wave (extended in space) and other times as a particle (not extended in space), but never both at the same time.
- The wavelength is equal to hc/E where E is the energy of the particle, c is the round-trip speed of light, and h is Planck’s constant. Thus, lower energy particles have longer wavelengths.